Large-Scale Fabrication of Protein and DNA Nanoarrays
Introduction
Recent advances in nanofabrication technology have contributed to the investigation into the advantages and behavior, at nanoscale, of biomolecules and living cells. Traditional nanofabrication platforms and techniques (including photolithography, selective chemical modification and soft lithography) have been used to fabricate nanoscale biomolecular arrays. However, most of these lithographic processes were originally developed for electrical engineering and material science applications - so they were not biocompatible. More recently,Dip Pen Nanolithography® (DPN®) has been used to fabricate chemically-functional nanoarrays capable of binding biomolecules, but the deposition process is only compatible with gold coated substrates and the arrays cannot be viewed with the most popular biological imaging techniques.
DPN is an established method of nanofabrication in which materials are deposited onto a surface using a sharp tip. In addition to generating small feature sizes, DPN systems enable rapid and flexible design of feature geometry. Using a multiple-tip cantilever "pen" array, the fabrication process can be scaled up to pattern hundreds to thousands of features in relatively short periods of time.
Here we demonstrate the ability of DPN to fabricate ordered arrays of biomolecules over large areas by using a block copolymer carrier to print gold nanoclusters directly to specific locations on a glass or silicon oxide surface. This capability not only enables array deposition on substrates commonly used in biomedical sciences, but also provides for a 3rd dimensionality on the substrate – which is often critical to the study of cell–substrate interactions.
Advantages of Block Co-Polymer Carriers
A recent publication by Mirkin et. al. demonstrated the utility of using block co-polymers as carriers of gold ions. In their study, an aqueous solution of the block copolymer poly (2-vinyl pyridine-b-ethylene oxide) (P2VP-PEO) was used as a carrier to transport gold ions (AuCl4-) onto a silanized silicon oxide substrate.
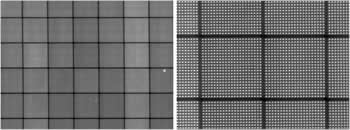
Figure 1. Dark field images of printed block copolymers demonstrate uniformity of patterning over large areas.
One of the advantages of using a polymer as a delivery matrix is that feature sizes much smaller than the original droplet size can be achieved; this is accomplished by removing the microdroplet's organic components with an oxygen plasma or heat treatment step. Working under low metal ion concentration conditions, feature size reductions of >90% (compared to print droplet size) are possible, resulting in highly uniform gold features as small as 50 nm in size.
Fabrication of Gold Nanoclusters
One of NanoInk's nanofabrication instruments, either the NLP 2000 System or the DPN 5000 System, can be used to deposit gold ions. These DPN platforms deliver X, Y, and Z positional accuracies of 50 nm and 1 nm, respectively, enabling the design and fabrication of arrays with arbitrary pitch and controllable feature sizes. A 12-"pen" tip cantilever array (M-type), which was used to pattern sub-micron sized droplets of a gold salt/P2VP-PEO mixture on SiO2 substrates (Figure 1). Once the polymer/ion mixture was coated on a cantilever, it behaved as a highly viscous liquid - beginning to flow at ~60% relative humidity; the higher the humidity, the higher the rate of material transport from tip to surface.
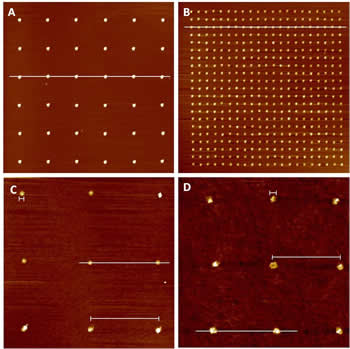
Figure 2. AFM images of various size gold nanoclusters remaining after polymer carrier removal. (A) Nanoclusters of 500 nm (B) Nanoclusters of 300 nm (C) Nanoclusters of 150 nm (D) Nanoclusters of 50 nm.
After polymer/ion mixture deposition, surfaces were heat treated to decompose the polymer and leave behind gold nanoclusters, as illustrated in Figure 2. Clusters were shown to adhere strongly to the surface and survived several subsequent rinsing and chemical reaction steps.
After heat treatment, arrays were rinsed with DI water to remove any remaining organic printing remnants. The arrays of gold nanoclusters were then ready to serve as biomolecular attachment points.
Using Nanoclusters to Capture Biomolecules
Regular arrays of gold nanoclusters are capable of capturing any thiol-modified biomolecule, but in this study we concentrated on binding thiol modified DNA and thiolated biotin.
In each case, a dilute solution of thiolated molecule was pipetted over the top of the gold nanocluster arrays. After 1 hour, slides were washed, blocked with a PEG-silane molecule, washed again, and then immersed in a dilute solution of complementary molecule (either DNA strands complementary to the previously-bound thiolated molecule – labeled with a 3' Alexa Fluor 547 tag - or Alexa Fluor 547-labeled streptavidin.)
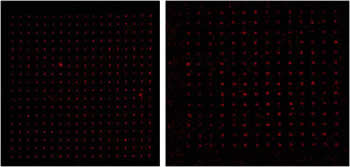
Figure 3. Fluorescent images of biomolecules attached to gold nanoclusters. (Left) Thiolated biotin bound to gold features and labeled with Alexa Fluor 547-tagged streptavidin (Right) Thiolated DNA bound to gold features, detected with a complementary strand tagged with Alexa Fluor 547.
Figure 3 shows that complementary molecules are present at each gold nanocluster position. Additional experiments showed that it was necessary to first expose gold nanoclusters to thiolated biomolecules in order to generate an observable fluorescence signal; this confirmed that biomolecular adhesion to gold clusters was the result of a chemical, and not a physical, bond.
Conclusion
We have demonstrated that NanoInk's powerful DPN systems can be employed to create nanoscale arrays of DNA or proteins by first creating binding sites capable of attaching to thiolated biomolecules. The biocompatible DPN deposition process can be easily scaled up to print large biomolecular arrays of arbitrary dimensions.
Reference
J. Chai, F. Huo, Z. Zheng, L. R. Giam, W. Shim, and C. A. Mirkin PNAS 2010, 107, 20202.
No comments:
Post a Comment